
Synthetic Biology: The Last Frontier
This article is a follow-up to the blog post I published on Substack. The Substack piece talks about the PR problem of Synthetic Biology, while this one is more technical for my nerdy friends on here. Enjoy!
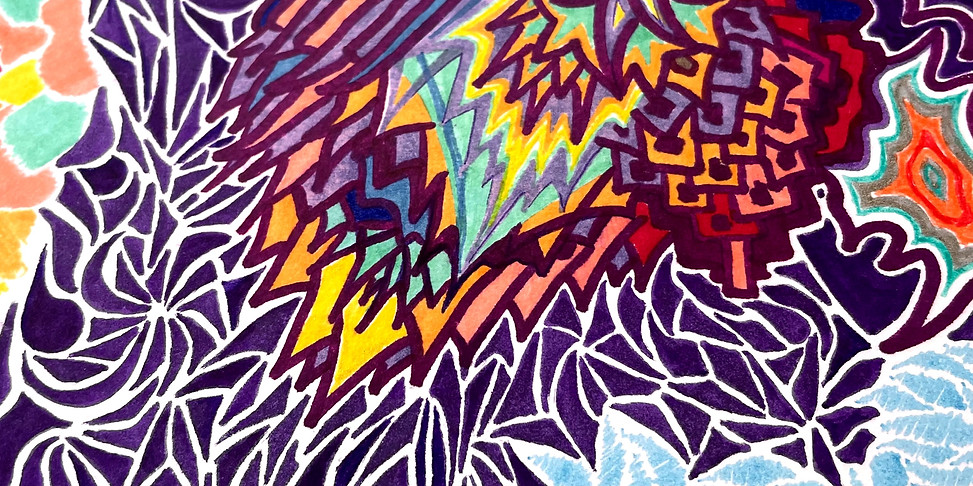
Humans have an innate drive for exploration. Our ancestors crossed thousands of miles on foot to get from Asia to the tip of South America. They reached isolated volcanic islands in canoes long before we even had the navigational tools to suggest that there is dry land in the vast emptiness of the ocean. We have conquered the great seas, mountains, deep forests, and endless deserts. We have even put out our probing telescopes into space, pointed at the places so remote that we would have to gear up for multi-generational expeditions to reach them. It seems that we have fully surveyed our world and learned everything there is to know about the universe.
But now we are turning inward – instead of expanding the reach of human activity to intergalactic frontiers, we are focusing on the microcosm within our cells. Opposite to Galileo’s telescope, our lenses are focused on the things exponentially small. And some of the things we are studying are too small to even see with a microscope: the molecules that make up our cells, like DNA and proteins, are smaller than the wavelength of light, so trying to probe their shape with a beam of light is like using a crane to build a house from Legos. We, molecular biologists, are using a different set of tools to probe living things.
Why are we doing this? One reason is that we are trying to answer the most basic question: “What is life?” by breaking it down to the smallest, simplest elements, and figuring out how they work. Once we figure out what life is, the next question is “Can we rationally engineer it?”. The second reason for understanding the fundamental workings of biology is to harness its power. Over millennia people have used biology for everything from food to building materials to medicines. But now we are expanding the capabilities of biological systems beyond what exists in nature – by venturing into the realm of synthetic biology.
Over the last century, we have learned enough about biology to understand the basic principles of life. In fact, we have learned so much that we can now alter the genetic makeup and physical characteristics of living things. This is commonly referred to as “synthetic biology”. The name probably brings to mind the image of cyborgs or artificial organs, but in fact, synthetic biology currently deals with things unseen to the naked eye – like bacteria and fungi. These simple organisms are a lot easier to study, yet tremendously useful. Plus they can teach us a lot about what life is – and how we can harness it.
Humans have been using domesticated microbes for thousands of years: we use yeast to brew beer and make bread and bacteria to ferment cheese and sauerkraut. In a similar process, scientists now use these microbes to make biofuels, specialty chemicals, and even materials. The difference is – up until now we have mostly relied on finding naturally occurring bacterial or yeast strains that make useful products, like alcohol. The job of synthetic biologists is to engineer microbes – to edit their genetic makeup, which in turn changes their physical expression – in a rational way in order to expand the repertoire of their products beyond what they can naturally make.
The synthetic manipulation of organisms starts from rewriting – or editing – the DNA code, the programming language of life. That means designing genetic sequences on a computer and then synthesizing them in the physical form. The process of DNA synthesis is kind of like putting beads on a string, except the beads are the four letters of the DNA code. Then, that DNA sequence, which holds instructions for making proteins, is inserted into cells that can “express” those proteins. Proteins can be thought of as tiny biological machines that can perform many functions, from spinning the microscopic “turbines” that provide the energy for the cell to building complex bioactive molecules that we use as medicines, fragrances, food flavors or cosmetic ingredients.
This process of genetic engineering is meticulously repeated, until the small tweaks in the microbe’s genetic code result in the desired output, such as making copious amounts of a useful product. And that is pretty much the goal of biomanufacturing: utilizing microorganisms to produce the stuff we use in our daily lives. But why do we want to go through this complicated process? Don’t we already have everything we need – things provided by nature or made from petroleum-based sources?
It's true: nature is a cornucopia of treasures. Plants, for example, are full of vitamins, antioxidants, delightful flavors, and even healing compounds that we have utilized over millennia. The problem is that to sustain the growing population of our planet, we need more and more land to provide us with enough of these things for everyone. Besides, many of the plants that contain important ingredients grow very slowly.
For example, to obtain vanilla extract from the Madagascar vanilla orchids, the plants need to grow for 3 years before they produce a flower, which has to be pollinated by hand, and then takes another 8-9 months to produce a mature vanilla bean. So, each tablespoon of extract, made from one whole vanilla bean, takes nearly 4 years to get. That’s a long time to wait just to make a cupcake!
What you can do though is take the genes from the vanilla orchid and insert them into yeast to make the same compound (called vanillin) that gives vanilla beans their sweet flavor – but in just a few days and much larger amounts – using yeast fermentation. You could also engineer these microorganisms to make things that we currently make out of oil. Everyone knows that oil is a finite resource; so, if we don’t want to give up the things made out of it, we need more sustainable alternatives – like products made with microbial fermentation that use sugarcane as a renewable, fast-growing source material to grow on.
Another important aspect of synthetic biology – besides transferring genes from one organism to another – is being able to control the expression of those foreign genes. People have long observed that microbes respond to their environment: for example, by changing the flavor profile of fermented beverages depending on the temperature or other conditions of the process. This gave scientists an idea to use that system like a switch to turn genes on and off when needed. This, I would say, is what really distinguishes synthetic biology from plain old genetic engineering.
While genetically engineered organisms (GMOs) have been used for a while now, synthetic biology is very new. And it’s that ability to not just edit, but control the behavior of living cells on demand, which makes it cool. For example, you could grow a yeast strain in a vat pretty fast and then turn on its special-chemical-making ability to direct all the output from millions and billions of cells to making that hard-to-make product. You could also make it change color in the presence of a certain chemical – either to alert you that the fermentation needs more nutrients or to detect harmful chemicals in the environment.
The ultimate goal of synthetic biologists is to make biology more like an engineering discipline, rather than an experimental art. Where if you know what you want to make – say, an antibiotic compound – you can go into software, design the genes and genetic switches (or other standard biological parts), synthesize that DNA and have it work like a charm the first time. We are still quite far from that owing to the immense complexity of biology. Life was not designed by an engineer; it evolved randomly through trial and error, and therefore each organism’s genetic code is so so so complex that the changes we make can have an unpredictable effect.
The desire to reduce the complexity of biological systems led to the creation of the minimal genome, one that contains only the most essential elements for life. This minimal genome was made completely from scratch, using the same process of synthesizing DNA like beads on a string and then tying those strings together to make one really long DNA ribbon. It was then inserted into a cell that had its own DNA destroyed and showed that the minimal genome was indeed enough to sustain the cell’s replication and function – to sustain life. In theory, you could take that minimal genome and customize it to your liking – like you would do if you were building a car from different parts.
Now that we have enough knowledge of what the essential elements of life are, the ability to design DNA sequences on the computer and “print” those instructions using DNA synthesis, then insert them into an empty chassis of a cell – we could design any organism we wanted. It’s not quite creating life from scratch. It’s more like Stable Diffusion or another type of AI-generated image: it takes something that exists in nature, changes it, and spits out something new and unique. It might not be super realistic, but it will be alive, and through experimentation, we could arrive at something really cool.
It may sound a bit like scientists are playing God, and in many ways they are. There is no way to fully anticipate all the unintended consequences. We are kind of shooting in the dark – thinking we can engineer biology only to be reminded that it’s too complex for our current understanding. The process can be a bit chaotic. But so is Nature. The perceived order, beauty and intelligent design we see in Nature is the result of random experimentation. There are perhaps rules that govern the structure of all things – certain patterns and ratios that are repeated throughout universe across all scales, from molecules to galaxies. And if there are, we are unlikely to be able to break them. So, whatever new life forms we may create, we will soon be able to get used to our new neighbors.
Thanks for reading, friends! If you liked this piece,
check out some of the other articles on this blog and sign up for my Substack!

Is there anything that can't be solved with CRISPR? From live cell imaging to COVID diagnostics, this biotech tool can do a lot more than just edit DNA.

Biotechnology is developing at an accelerating pace. Here is a list of 10 crazy Sci-Fi ideas that are likely to become a reality by 2030.